Research
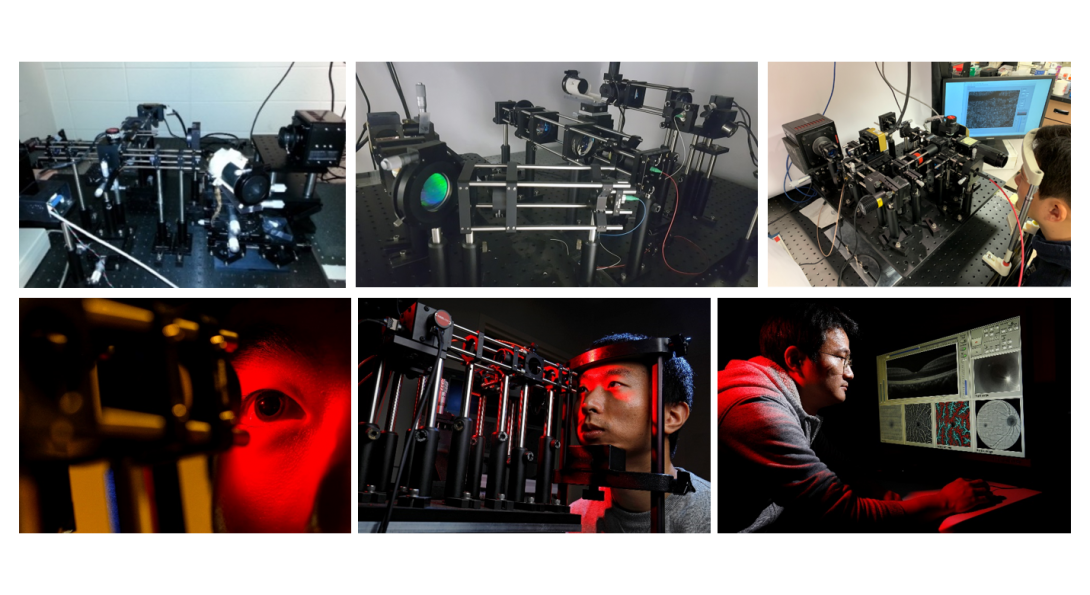
Our lab is focusing on advanced ophthalmic instrumentation and quantitative imaging technology to advance retinal study, disease diagnosis and treatment evaluation. Our current research includes wide field fundus photography, functional optical coherence tomography (OCT), quantitative OCT angiography (OCTA), super-resolution ophthalmoscopy, functional imaging of neurovascular coupling, machine learning based image analysis and AI classification.
Wide field fundus photography Heading link
Wide field fundus photography is essential for remote screening, diagnosis, and treatment evaluation of eye diseases. However, the complexity of conventional transpupillary illumination and imaging mechanisms has made it technically challenging to construct a wide field fundus camera. To overcome this challenge, we have developed ultra-widefield fundus cameras that use trans-palpebral and trans-pars-planar illumination techniques. Additionally, we have developed a novel miniaturized indirect ophthalmoscopy approach for wide field fundus photography. By further developing low-cost and easy-to-use widefield fundus photography, we can offer an affordable solution for telemedicine assessment of eye diseases, thereby improving access to eye care for patients in rural and underserved areas. Our team of experienced researchers is dedicated to using the latest technology and cutting-edge techniques to develop and refine wide field fundus photography for both adult and pediatric patients.
Wide field fundus Image 1 Heading link
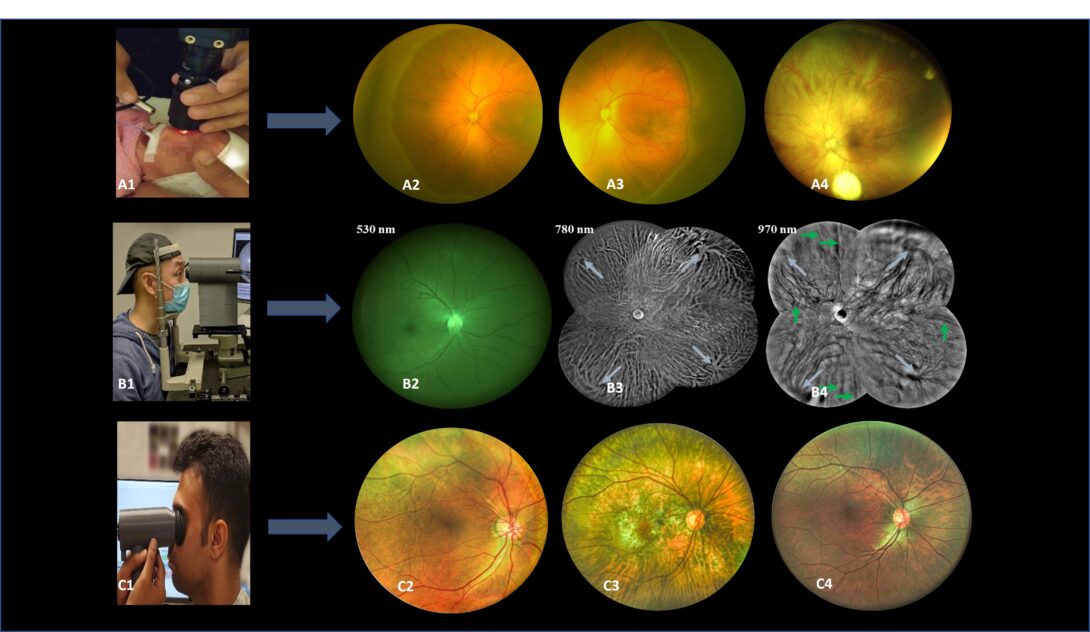
Figure1 A1. Pediatric fundus camera during imaging. A2-A4. Sample images from pediatric fundus camera. B1. Trans eyelid fundus camera during imaging. B2-B4. Sample images with different light wavelengths from the trans-eyelid fundus system. C1. Miniaturized HDR trans pupillary fundus camera during imaging. C2-C4. Sample HDR images from trans pupillary fundus system.
Quantitative OCTA analysis and AI classification of eye diseases Heading link
The retina, located at the posterior part of the eye, is responsible for converting light into bioelectrical signals and transmitting them to the central nervous system. As a component of the CNS, the retina is composed of multiple layers of neural and vascular tissue, making it vulnerable to systemic conditions like diabetes and sickle cell anemia. These diseases can lead to diabetic retinopathy (DR) and sickle cell retinopathy (SCR), which often remain asymptomatic until irreversible damage occurs. Early detection and intervention are critical for preventing or slowing down disease progression. To visualize retinal changes and diagnose these diseases, various retinal imaging techniques such as fundus photography, optical coherence tomography (OCT), and OCT angiography (OCTA) are used. Fundus photography is a quick and relatively inexpensive technique, but it has limited resolution to visualize microcapillary changes. OCT provides high-resolution 3D cross-sectional information to visualize individual retinal layers. OCTA provides high-resolution visualization of the retina vasculature. However, current screening methods rely on manual grading by trained graders, leading to grading errors. Thus, developing quantitative features is crucial for reliable diagnosis.
Image Quantitative OCTA Analysis Heading link
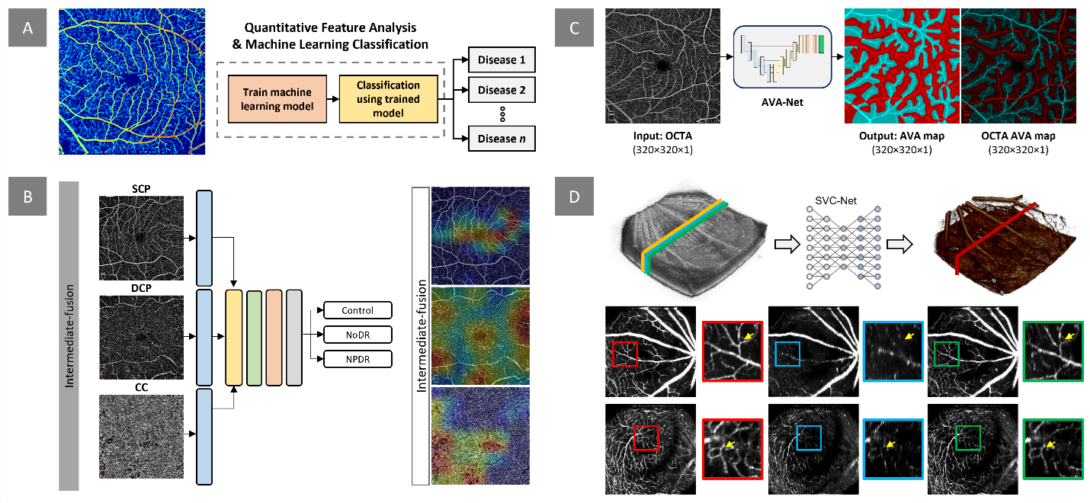
Figure. Our research project on quantitative OCTA analysis and AI classification focuses on several areas. A) We aim to develop digital biomarkers for early disease detection and evaluation of treatment outcomes. This example demonstrates the measurement of normalized blood flow index, which can be used to train machine learning models for disease detection and staging. B) In addition to extracting quantitative features, we design and implement deep learning models for automated classification of retinopathy using OCTA images. Our recent work investigates the effects of multi-layer OCTA fusion for robust diabetic retinopathy classification. C) Clinical observations indicate that retinopathies affect arteries and veins differently. To differentiate between different retinopathy stages, we employ differential artery-vein (AV) analysis. Our recent work explores deep learning-based AV-Area (AVA) segmentation for this purpose. D) Traditional OCTA processing requires repeated scans, leading to increased acquisition time and potential motion artifacts. To address this, we propose a deep learning approach that utilizes the spatial vascular connectivity (SVC) between scans in a single-scan volumetric OCT for OCTA prediction. Overall, our research project combines quantitative OCTA analysis and AI classification techniques to advance the field of retinopathy detection and characterization.
Functional OCT of retinal neurovascular coupling Heading link
Retinal neurodegenerative diseases, including age-related macular degeneration (AMD), retinitis pigmentosa (RP), diabetic retinopathy (DR), and glaucoma, can cause significant vision loss without timely medical interventions. Moreover, as part of the central nervous system (CNS), the retina is also affected by other neurodegenerative diseases, such as Parkinson’s and Alzheimer’s diseases, which are the primary causes of dementia. Early detection of these conditions is crucial for studying and developing preventive strategies. Functional imaging of neurovascular coupling defects promises early detection of neurodegeneration, but direct access to the brain for high-resolution examination is challenging. The retina provides a unique window for high-resolution study of neurovascular coupling defects. Recent advances in functional optical coherence tomography (OCT) have enabled imaging of transient intrinsic optical signal (IOS) changes correlated with stimulus-activated retinal neural activity and hemodynamic response. Functional OCT of neurovascular coupling holds the potential for high spatiotemporal resolution methodology to investigate coherent interactions between neural activities and hemodynamic changes in the retina, offering a promising tool for early detection and better management of neurodegenerative diseases.
Functional OCT image Heading link
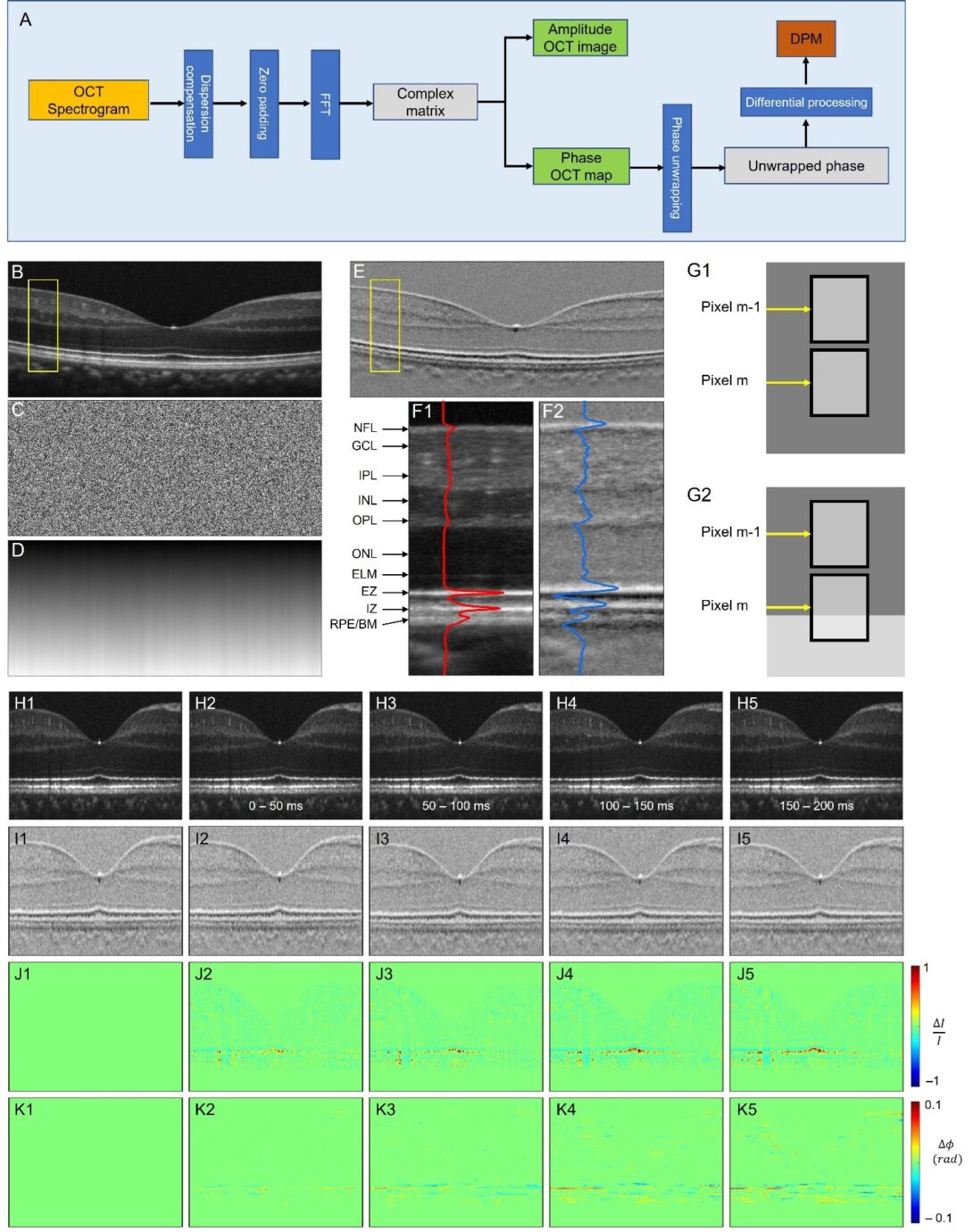
Fig. 1. Differential phase mapping (DPM) and DPM IOS. (A) A flow chart of amplitude OCT and differential phase mapping (DPM) processing. Representative OCT amplitude image (B), corresponding phase map (C), unwrapped phase map (D), and DPM (E). (F) Flattened amplitude (F1) and DPM (F2) images corresponding to the yellow rectangular areas in (B) and (E). The blue and red curves show the waveform profiles of the amplitude image and DPM. (G) Schematic diagram of differential phase interpretation. (H) Amplitude image sequence. (I) DPM sequence. (J) Amplitude-IOS sequence. (K) Phase-IOS sequence. For more details, please see here.
Super-resolution scanning laser microscopy and ophthalmoscopy Heading link
High-resolution microscopy is crucial for accurate diagnosis of medical diseases and has revolutionized the field of biological sciences. However, conventional microscopes have a light diffraction limit that limits their spatial resolution. Structured illumination has been explored to overcome this limit in wide field light microscopy, but practical deployment of structured illumination microscopy (SIM) for in vivo retinal imaging is often challenging due to phase errors caused by eye movement. To address this, our team developed super-resolution scanning laser microscopy using virtually structured detection (VSD), which provides an easy, low-cost, and phase-artifact free strategy to achieve super-resolution in scanning laser microscopy. We have demonstrated the feasibility of in vivo super-resolution scanning laser ophthalmoscopy (SLO) by combining a line-scanning strategy. Our ongoing research investigates the potential of using VSD-based SLO for in vivo super-resolution functional imaging of human photoreceptors, with significant implications for improving the diagnosis and treatment of retinal diseases.
Super-resolution scanning laser microscopy and ophthalmoscopy Heading link
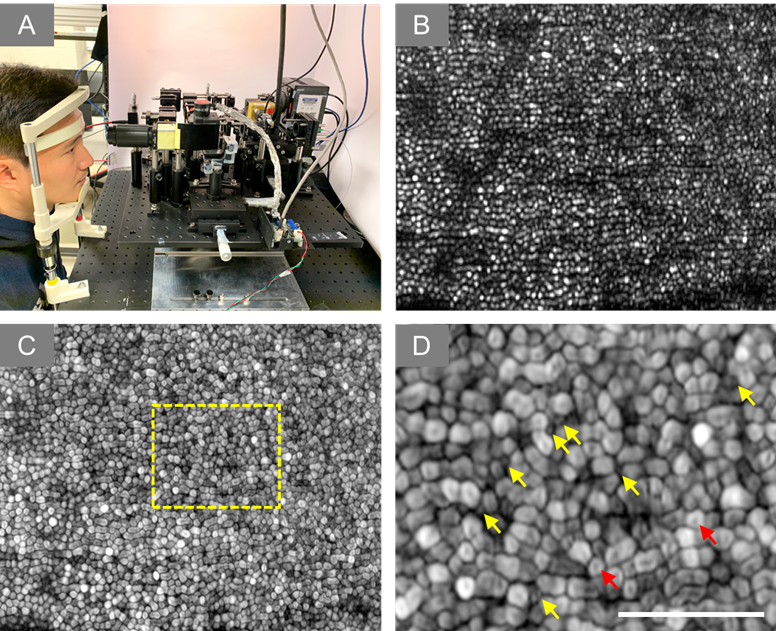
Figure 4. (A) Experiment setup of super-resolution SLO. (B) Representative image of human retina. (C) Dynamic motility analysis of retinal photoreceptors. (D) Enlarged illustration of the yellow window in C. The yellow and red arrowheads point to individual rod and cone photoreceptors, respectively.
Active grants: Heading link
Nonmydriatic Ultra-Widefield Fundus Photography Employing Trans-Pars-Planar Illumination
Source: NIH/NEI R01
Functional tomography of neurovascular coupling interactions in healthy and diseased retinas
Source: NIH/NEI R01
A portable ultra-widefield fundus camera for nonmydriatic examination of retinopathy of prematurity
Source: NIH/NEI R43
Functional imaging of retinal photoreceptors
Source: NIH/NEI R01
Development and validation of a holographic waveguide-based oculometer for objective and comprehensive oculomotor disorder assessment
Source: NIH/NEI R21
INSTRUMENT SHOP CORE
Source: NIH/NEI P30
Completed projects: Heading link
Super-resolution ophthalmoscopy for in vivo retinal imaging
Source: NIH/NEI R01
Simultaneous imaging of photoreceptor and post-photoreceptor responses in the retina
Source: NSF CAREER
Angle-resolved polarization signal imaging of early receptor potential
Source: NIH/NIBIB R21
Multifocal Optical Coherence Microscope
Source: NIH/NCRR R21
Concurrent Structural and Functional Imaging of Retinal Neurons
Source: DANA Foundation (Brain-Immuno Imaging program)
Development of a Microlens Array Confocal Ophthalmoscope
Source: Eyesight Foundation of Alabama